Basel lies 251km due northeast by road from Geneva, putting a precise Swiss measure on the distance between big finance and big science.
Headquartered in the Geneva suburb of Meryin, CERN1 has served as the global mecca of particle physics research since it switched on the Large Hadron Collider (LHC) in 2008.
Via the LHC – the biggest and most expensive scientific instrument ever built – particle physicists have probed the deepest secrets of the universe, producing a series of stunning results along the way.
Yet despite throwing about 1.3 terawatts of energy every year at the smallest known components of reality, CERN scientists have not to date been able to smash the longstanding fundamental theory of physics known as the ‘standard model’.
Basel IV: A theory of everything
Arguably, the Basel-based Bank of International Settlements (BIS) has been met with a similar challenge in establishing a ‘standard model’ of banking.
Founded amid a worldwide financial meltdown in 1929 as a central bank collective for developed countries – initially to manage World War I reparations – the BIS took until 1988 to reach agreement on an overarching risk management model for the global banking system: the Basel Accord, or Basel I, established core bank capital rules that were updated in a 2007 sequel.
The limitations of Basel II, however, emerged almost as soon as the ink was dry during a global financial crisis that blew holes in bank capital assumptions. Slated originally for a start date in 2013, Basel III implementation was delayed a decade until January last year.
In 2017 the BIS introduced a Basel III update, variously known as ‘Finalising post-crisis reforms’, Basel 3.1, Basel ‘Endgame’ or Basel IV.
Has the BIS finally cracked the deepest secrets of the global banking system with Basel IV? History suggests a ‘standard model’ of risk management in finance is probably more elusive than the more than five decades old physics theory (of almost everything) that has survived all attempts to debunk it.
Forces and particles: putting the pieces together
Pioneered by Nobel Prize-winning US physicist Steve Weinberg in early 1970s, the standard model provides a logical framework for how the basic building blocks of the universe, a complex array of particles, interact with three of the four known fundamental forces.
Weinberg completed a puzzle that had occupied physicists for decades, building on previous work such as the ‘eightfold-way’ particle classification system developed by another Nobel laureate, Murray Gell-Mann in the early 1960s.
The standard model has successfully explained almost all experimental results and precisely predicted a wide variety of phenomena, capped off by the LHC-led discovery of the Higgs boson at CERN in 2012.
Under the model, a class of bosons known as gauge bosons act as ‘force carriers’ between particles. Gauge bosons include the photon (carrying the electromagnetic force), gluon (the strong nuclear force), and W and Z bosons (carry the weak nuclear force).
The model also explains quarks, the fundamental, indestructible particles that combine to form the composite fermions, protons, and neutrons as illustrated below.
We say the standard model has successfully explained almost all experimental results because there is one obvious hold-out: gravity – that most fundamental of forces – has, to date, resisted being drawn into the orbit of the standard model.2
While some theories suggest a fifth fundamental force might lie outside the current standard model, the evidence, from CERN and elsewhere, leaves us stuck with four for now.
Figure 1: The standard model
High-energy: Bank risk-weighting fundamentals
Just as quarks function as the unbreakable components in the standard model of high-energy physics, banking supervision also rests on fundamental risk factors.
In bank governance models, a measure of risk-weighted assets (RWA) underpins the risk-based capital (RBC) metric that determines lending limits for institutions.
According to the calculation method, RWAs act as denominator in the RBC equation with eligible capital (essentially, common equity shares) in the numerator role.
Capital ratios are an important indicator of the solidity of a financial institution, playing a key role in determining whether the supervisor will approve bank balance sheet management plans, such as dividend payouts or share buy-backs.
Simply put, banks arrive at RWA measures by multiplying the exposure amount by the relevant risk weight for the type of loan or asset in question – repeating the calculation across all loans and assets to come up with a figure representing total credit risk-weighted capital for the institution as a whole.
The exact formula is more intimidating, as below:
Figure 2: An intimidating formula
But while the standard model hinges on metrics tightly constrained by physical reality, the banking counterpart has more wiggle room in the measurement department.
Banks typically assess RWAs in two ways: the advanced internal ratings-based (A-IRB) and foundation internal ratings-based (F-IRB) approaches.
Subject to certain constraints, banks using the IRB methods can rely on internal models as inputs to determine regulatory capital requirements for credit risk.
RWA variance among banks reflects a range of factors including business models and asset mixes but risk calculation methodology and modelling inputs can also skew the score, rendering like-for-like comparison difficult for both regulators and investors.
Basel IV should help iron out some of these RWA-based inconsistencies – in Europe at least where the new rules will introduce an output floor in capital requirements calculated under internal models at 72.5%.3
Across the Atlantic, US regulatory agencies pushing for implementation of the Basel ‘Endgame’ rules to increase the strength and resilience of the financial system have met with strong resistance from banks and some congressmen.4
The global standard banking model, clearly, has some distance to travel.
Conclusion
Particle physics, as witnessed by the success of the standard model, has come a long way.
But the model remains incomplete despite the failure of the LHC to poke holes in the theory: CERN has proposed a new €20bn machine, three-times the size of the current collider, that might do the job (targeted launch date in the 2070s).
Now at its fourth iteration, the latest Basel bank risk management regime, by contrast, remains more theory than practice.
As the updated rules come into play will we gain a clearer picture of the risks in banking?
Will that knowledge prevent a future crisis?
And, perhaps more importantly for the global financial system, will the US be on board?
The story of how the standard model of particle physics was assembled and the mathematical principles underlying it might provide some clues.
But that’s for another Fiorino.
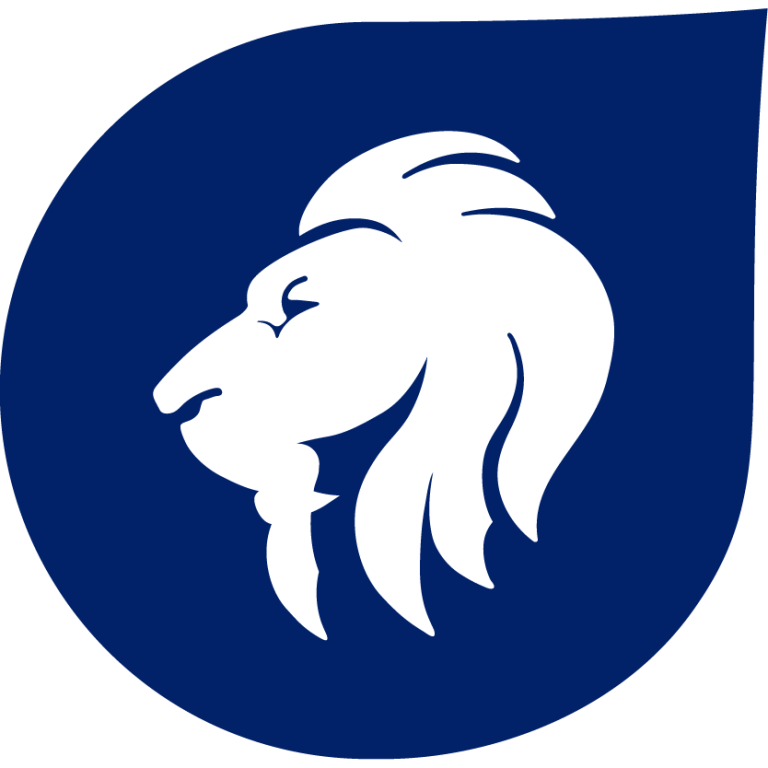
Nobel laureate Isidor Isaac Rabi (1898-1988) in a quipping mood when learning of the discovery of the muon particle in 1934.*
1 Conseil Européen pour la Recherche Nucléaire (CERN) is an intergovernmental organization that operates the largest particle physics laboratory in the world. Established in 1954, it is based in Meyrin, western suburb of Geneva, on the France–Switzerland border.
2 Although, admittedly, the theory does predict the yet-to-be-discovered force-carrying ‘graviton’ particle.
3 See: https://www.bis.org/basel_framework/chapter/RBC/20.htm?inforce=20280101&published=20201126
4 See: https://www.federalreserve.gov/newsevents/pressreleases/bcreg20231020a.htm
*The muon is one of the fundamental subatomic particles, the most basic building blocks of the universe as described in the Standard Model of particle physics. Muons are similar to electrons but weigh more than 207 times as much. The muon is part of the lepton group.
For more from our Unconstrained Credit team, explore our capabilities page.